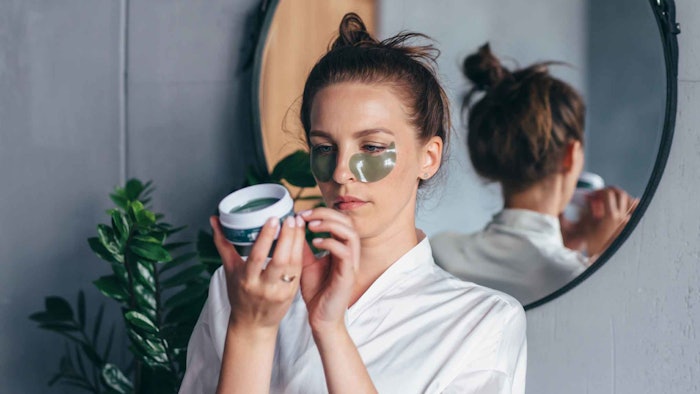
Consumers are drawn to products that promise sustainability by minimizing environmental impact. In fact, this influences their purchasing decisions. In France, for example, three out of four consumers report they are changing their daily practices to reduce the impact of their consumption.1
Log in to view the full article
Consumers are drawn to products that promise sustainability by minimizing environmental impact. In fact, this influences their purchasing decisions. In France, for example, three out of four consumers report they are changing their daily practices to reduce the impact of their consumption.1
Among the sustainability claims marketed, biodegradable has been found to be the most popular and persuasive, with 74% of shoppers saying this would encourage them to purchase a product.2 Manufacturers’ response to this demand has been reflected in ambitious sustainability initiatives and the steady increase of new product launches featuring biodegradable claims.
Notably, this claim comes with the responsibility for manufacturers to prove that the product is in fact biodegradable. Unfortunately, with the rush to capitalize on this changing consumer behavior, the potential for greenwashing or misleading advertising is high if due diligence is not performed.
In 2020, the European Commission sampled a range of environmental claims and found that 53% of them provided vague or misleading information.3 Greenwashing has already prompted the French government in 2023 to ban the claims biodegradable, environmentally friendly or any other equivalent wording on a product or packaging.4 This issue, along with others, has also led to a proposed EU-wide policy restricting and harmonizing green claims.
So, what does biodegradable actually mean? There are many misconceptions around biodegradability, even among those involved in the development, substantiation and communication of a biodegradable product. This stretches from R&D and regulatory affairs experts to marketeers, all of whom have a different degree of understanding of the term.
This article aims to highlight the fact that there are variations in how even industry experts understand this term. It will elucidate gaps in understanding by introducing the science behind biodegradability, explaining common terminology and clarifying the limitations of biodegradation tests. Ultimately, the goal is to help those vested better understand biodegradability and ask the right questions.
Defining and Calculating Biodegradability
Biodegradability is a material property based on the measurement of biodegradation, which is the process by which living things break down organic matter. An organic material (i.e., carbon-containing) is considered biodegradable if over time, it is completely broken down to harmless byproducts by microorganisms such as fungi and bacteria in the environment.
The process of complete biodegradation may also be referred to as ultimate biodegradation or mineralization. Biodegradation may occur in the presence (aerobically) or absence of oxygen (anaerobically), wherever there are living microorganisms (e.g., soil, sediment, water, etc.).
In aerobic environments, microbes use carbon in an organic material as a food source for growth and cellular maintenance (e.g., to form new cellular matter) and, in turn, consume oxygen (O2) and respire carbon dioxide (CO2). Given a known amount of organic carbon and its elemental composition, it is possible to calculate the theoretical maximum amount of oxygen required and carbon dioxide produced to consider the material to be completely broken down.
For example, 1-octanol with a molecular formula of CH3(CH2)7OH and a molecular mass of 130.23 g/mol has 73.78% carbon content. Assuming 100% mineralization of 1-octanol added at a rate of 10 mg, the theoretical maximum degradation measured as inorganic carbon (ThIC) is equal to the amount of carbon from 1-octanol added at the start of the test, or 7.37 mg of carbon (73.78% of 10 mg).
By comparing these theoretical values to measurements of oxygen and carbon dioxide over time and subtracting the oxygen consumed or carbon dioxide produced from the blank (i.e., the background noise from microbes not exposed to the test substance), it is possible to determine the extent and rate at which an organic material has degraded. The result of the measurement compared to the theoretical maxima is expressed as a percentage at a point in time.
An example equation is provided for interpreting degradation based on carbon dioxide, measured as inorganic carbon:
Dt = [ΤΙCt - ΤΙCB]/TOC x 100
Where Dt = % degradation at time t; TICt = mg TIC (total inorganic carbon) in test chamber at time t; TICB = mean mg TIC in blank control replicates at time t; and TOC = mg TOC (total organic carbon) added initially to the test chamber
This is the driving principle behind the common test standards used for measuring biodegradation.
Proving Biodegradability
Having established a working definition of biodegradability and explaining how it can be measured, it is important to next determine what is considered proof of biodegradability. The definition itself provides some hints, though they are still somewhat vague and may leave more questions than answers. Fortunately, the industry has gained experience from decades of evaluating biodegradability – and learning from mistakes along the way. Following are five of the most critical aspects of proving biodegradability.
1. Standard, specification or certification: At the top of the list, a claim of biodegradability should always be backed by measured data from an appropriate test standard, specification or certification body. Standards, by design, have been developed to guide the user to obtaining valid and reproducible test data under conditions within a specified range. Certain standards are designed for specific classes of chemicals or products, or to represent biodegradation in specific media (e.g., soil or water).
This has led to dozens of available standards and the appropriate standard should be selected for the product and circumstances. Examples of standardization bodies include the Organization for Economic Cooperation and Development (OECD), the American Society for Testing and Materials (ASTM) and the International Organization for Standardization (ISO).5-7
Though some standards have associated criteria (e.g., timeframe and threshold of degradation) for interpreting data to conclude biodegradability (e.g., OECD 301/310 Test for Ready Biodegradability), other standards do not (e.g., OECD 302 Test for Inherent Biodegradability).8 This is where specifications and certifications typically go further than test standards in that they assist with test interpretation for biodegradability conclusions and, in the case of certifications, involve review by third-party agencies.
An example of a specification offered by ASTM is D6868, the “Specification for Biodegradable Plastics Used as Coatings.” An example of a certification body is the TUV group (Technical Inspection Association). However, most specifications and certifications have been developed to support claims of plastic and packaging products and not the chemicals they may contain. Other examples of certifications that may assess biodegradability, among other aspects, include government certifications such as the European Union Ecolabel.
2. The environment of disposal: The supporting biodegradation data accompanying a claim should be obtained from test standards that best represent the conditions under which the product will primarily be disposed. For example, for water-soluble chemicals released down the drain to wastewater, aquatic biodegradation tests such as the OECD 301/310 are appropriate. Evaluating and specifying the environment where the product will be disposed is critical, as different environments have different conditions (temperature, oxygen, nutrients, etc.) that influence microbial communities – determining whether biodegradation occurs and at what rate it proceeds.
These differences become clear by, for example, comparing a compost environment to a soil environment. Composting environments tend to have elevated temperatures (e.g., ~50⁰C), high moisture and nutrients. Soil conditions tend to be lower in moisture and temperature (e.g., ~20⁰C) and in some cases, nutrient-poor. This leads to different microbial communities, densities and activities and therefore the capacity to degrade. In the case where a material needs a thermal trigger to initiate biodegradation, which may only be found in composting, these materials may not degrade in soil at all.
3. Evidence of complete degradation: The definition of biodegradability includes the phrase “completely broken down” and the biodegradation data should indicate this to the extent practical. Complete degradation means that the carbon in the product is completely utilized by the microbes, resulting in microbial biomass (i.e., cellular matter), evolved CO2 (under aerobic conditions) and evolved CO2 + CH4 (under anaerobic conditions).
Disintegration, weight loss and partial or primary degradation (i.e., oxidation to chemical intermediates) are not generally considered acceptable evidence for concluding a material is biodegradable. In practice, there are limits to the abilities to measure complete degradation in experimental studies because the cost and time required for such precise studies (e.g., radiolabeling techniques) is high.
Standard test methods such as those used to assess chemicals (e.g., OECD 301/310 Test for Ready Biodegradability) measure CO2 evolution directly, or indirectly via oxygen consumption or dissolved organic carbon. These standards use degradation thresholds of 60-70% as evidence of complete degradation. The remaining 30-40% of the carbon balance not accounted for is assumed to be assimilated as microbial biomass (i.e., new cellular matter, as mentioned previously; see the hypothetical example in Figure 1).
Figure 1. Hypothetical example of an idealized biodegradation curve demonstrating complete degradation
Other methods such as those designed to assess plastics and packaging materials (e.g., ASTM and ISO standards) may use higher thresholds or thresholds that are interpreted relative to a reference material (such as natural compounds like cellulose). These follow the same principle.
4. Specification of time: The time or duration required for complete degradation should also be a standard component of the data, since it is as important as the extent of degradation. If something completely degrades but it takes a very long time, this may be considered misleading and unacceptable.
The amount of time considered acceptable for degradation depends on the product in question. The timeframes observed in standards range from 28 days up to 180 days or more. The reason for the large range is that some materials are inherently more difficult to degrade; for example, a material comprised of insoluble plastic.
In other cases, the allowed time may be long because the material has an application period where it must withstand degradation to do its intended function. Examples of these include agricultural mulching films or nutrient prills. In general, aquatic environments consider shorter times than soil or sediment in part due to the sensitivity of the ecosystems and the impact of environmental conditions on the expected rate of biodegradation.
5. The material tested: The form, purity and to some extent, the composition of the material tested should also be documented. Biodegradation tests should not be performed on formulas or chemical mixtures whose constituents could otherwise be isolated and tested individually. Ideally, biodegradation tests should be performed on pure, single-constituent materials but this is not always possible and in some cases, exceptions must be made (e.g., natural extracts that are mixtures; chemical surfactants and polymers that are intrinsically mixtures of like structures, etc.).
This is because biodegradation tests typically use non-specific measures of biodegradation, meaning the material itself is not directly quantified (such as oxygen or carbon dioxide). Testing chemical mixtures or low purity samples, for example, may result in false positives, as all organic components in a mixture may contribute to the biodegradation signal measured. An example of a false positive would be if one degradable constituent in a mixture was present at a high enough quantity, allowing the mixture to pass the threshold of the test (i.e., 60-70%) and thereby masking another constituent that does not degrade at all.
Limitations
It is important to understand that fully or completely biodegradable does not mean a measurement of 100% degradation (measured as CO2 evolution or O2 consumption) in a typical screening level biodegradation test, since in the short term (weeks to months), 100% isn’t likely for these analytes. This is because some of the carbon consumed is incorporated into microbial cells (as the microbes grow).
The carbon in the microbial cells is not readily available in the short term for degradation to be evolved to CO2. This step of the process takes much longer, as the carbon is less bioavailable (e.g., higher molecular weight, insoluble, etc.) and less energetically favorable for degradation. Eventually, the dead microbial cells are further degraded into highly oxidized biologically derived organic substances (e.g., humic acid) and these take years, to hundreds – or even thousands – of years to degrade to CO2. This slow degradation, however, creates organic-rich soils, sediments and fossil fuels.
So when something is described as being fully biodegradable, as indicated by one of the standard tests that measure CO2 evolution or O2 consumption, this means the carbon that was put into the test (i.e., the test substance) has completely degraded to CO2 and microbial biomass. This is also why the tests have passing thresholds for CO2 evolution or oxygen consumption that are, e.g., 60% of the theoretical maxima and not 100%.
As stated previously, the level of degradation considered as complete or fully degraded for a substance undergoing one of these tests will vary depending on their chemical composition, as this affects how the microbes will utilize them (in terms of mineralization to biomass carbon conversion ratio). As demonstrated experimentally in the 1970s and 1980s, typical mineralization to biomass carbon conversion ratios for chemicals are around 60/40, hence the basis for OECD passing thresholds of 60% CO2 evolution or O2 consumption, since biomass would not be accounted for directly by these analytes.
The 60% threshold for CO2/O2 does, however, coincide with ~100% dissolved organic carbon (DOC) removal that accounts for carbon converting to both CO2 and biomass (since biomass would not contribute to DOC levels) and hence, proof of concept and evidence of complete degradation, despite 100% of the theoretical maxima CO2 evolution or O2 consumption not being achieved. For OECD tests that measure DOC removal, while 100% degradation is possible, as mentioned, their pass thresholds are set to 70% for concluding complete biodegradability mainly to avoid being overly stringent because less degradation takes place in laboratory batch cultures than what would occur in the real world.
Implications for the Personal Care Industry
With a deeper definition of what constitutes biodegradability, key stakeholders in the industry are better equipped to understand claims and ask clearer, more specific questions about products; such as what method has been used to test for biodegradability. Aligned language, definitions and standards are a first important step in the journey toward transparency and authenticity, beginning at the crucial ingredient selection stage.
There is much work to be done to improve the understanding of biodegradability in personal care. This remains an area of active research and will continue evolving for years to come. While manufacturers bear the responsibility of substantiating biodegradability claims, they also have a unique opportunity to lead the way by adopting best practices and setting higher standards for the industry.
References
1. GreenFlex. (2022, Oct). GreenFlex-ADEME baromètre de la consommation responsable (GreenFlex-ADEME barometer of responsible consumption 2022). Available at https://www.greenflex.com/actualites/ressources/etude-barometre-consommation-responsable-2022/
2. Wright, G. (2020, Jan 29). 83% of shoppers mislead by green and sustainable advertising. Retail Gazette. Available at https://www.retailgazette.co.uk/blog/2020/01/83-of-shoppers-mislead-by-green-sustainable-advertising/
3. European Commission. (Accessed 2024, Feb 27). Environmental footprint methods. Available at https://ec.europa.eu/environment/eussd/smgp/pdf/2020_Greenclaims_inventory.zip
4. République Française. (2020, Feb 10). Article 13, LOI n° 2020-105 du 10 Février 2020 relative à la lutte contre le gaspillage et à l'économie circulaire (LAW no. 2020-105 of February 10, 2020, relating to the fight against waste and the circular economy (1). Legifrance. Available at https://www.legifrance.gouv.fr/jorf/id/JORFTEXT000041553759/
5. ISO. (Accessed 2024, Feb 27). Standards. Available at https://www.iso.org/standards.html
6. Hunt, K. (Accessed 2024, Feb 27). Standardization news. Standards for biodegradable plastics. ASTM International. Available at https://sn.astm.org/features/standards-biodegradable-plastics-ma19.html
7. OECD iLibrary. (Accessed 2024, Feb 27). OECD guidelines for the testing of chemicals, section 3. Available at https://www.oecd-ilibrary.org/environment/oecd-guidelines-for-the-testing-of-chemicals-section-3-degradation-and-accumulation_2074577x
8. OECD iLibrary. (Accessed 2024, Feb 27). Revised introduction to the OECD guidelines for testing of chemicals, section 3. Available at http://tinyurl.com/3x56tpk4; doi.org/10.1787/9789264030213-en