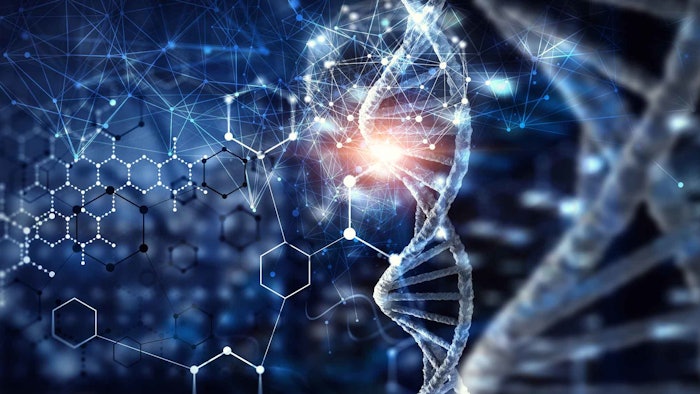
The Greek myth of Prometheus tells the tale of a titan who stole fire from the Olympian gods and granted it to humanity. From the fire came knowledge, power and liberty. Prometheus encapsulates the spirit of science: defiance against adversity, unbridled curiosity and the relentless resolve to redefine what is possible. Biotechnology eloquently mirrors the tale of Prometheus, as it fundamentally involves gaining control over the once-thought-divine biological systems that define us, as well as manipulating them to advance humanity.
Log in to view the full article
The Greek myth of Prometheus tells the tale of a titan who stole fire from the Olympian gods and granted it to humanity. From the fire came knowledge, power and liberty. Prometheus encapsulates the spirit of science: defiance against adversity, unbridled curiosity and the relentless resolve to redefine what is possible. Biotechnology eloquently mirrors the tale of Prometheus, as it fundamentally involves gaining control over the once-thought-divine biological systems that define us, as well as manipulating them to advance humanity.
The cosmetic industry is undergoing a biotech revolution to lead the next frontier in solutions to address skin aging and pathophysiology. Some of the many new technologies spearheading this revolution are stem cell engineering, archaeosomes and bacteriophages. This article describes the complexity of mechanisms in skin aging and how these next-gen biotechnologies are the path forward to democratize beauty.
Mechanisms in Skin Aging
Skin aging is a fascinatingly complex phenomenon that covers chronological factors and extrinsic factors, both of which lead to unique signaling changes across the entire dermal matrix. Inflammaging refers to the cyclical pattern of chronically elevated inflammation leading to accelerated aging, which then results in even more inflammation.1
This chronic inflammation is characterized by elevated levels of inflammatory cytokines (signaling molecules that regulate immune responses) such as Tumor Necrosis Factor alpha (TNFα) or Interleukin-6 (IL-6).1 These cytokines regulate innate immunity, which is the first line of defense against foreign invasions – and known to become dysfunctional with advanced aging (alongside more specific immune responses, termed active immunity).
As people age, the level of senescent cells (non-dividing cells) increases.1 Senescent cells secrete proinflammatory cytokines and thus contribute to inflammaging. All these factors elevate enzymes that degrade components of the extracellular matrix (e.g., collagen or elastin), exacerbate susceptibility to infections and gradually increase oxidative stress, among many other detrimental consequences (see Figure 1, below). Elucidation of these mechanisms is necessary to develop anti-aging actives and two common targets in modern anti-aging treatments are: nuclear factor kappa B (NF-ⲕB) and sirtuins.
NF-ⲕB
NF-ⲕB is a transcription factor and master regulator of the immune system.2 In the classical pathway (see Figure 2, below), the proteins p50 and p65 compose NF-ⲕB. Under normal conditions, NF-ⲕB is bound by IⲕB proteins (named inhibitor of NF-ⲕB) that inhibit NF-ⲕB translocation to the nucleus.
When a cell is under conditions of stress, such as oxidative stress, UV exposure or pathogen invasion, the NF-ⲕB inhibitor is disrupted by IKK, which frees NF-ⲕB.
Also in the classical pathway, cytokines (such as TNF⍺) bind to their respective receptors and initiate this NF-ⲕB activation process. Once free, NF-ⲕB can migrate to the nucleus and have substantial impacts on gene expression, such as increasing proinflammatory signals and attenuating the cell cycle (both of which have aging implications).2
NF-ⲕB can also be activated by other pathways and sources (termed alternative and atypical pathways in Figure 2, below), each of which presents opportunities where active ingredients may be effective. For example, an active may inhibit cytokine receptor activity; it might inhibit the deactivation of the IⲕB complex; or it might inhibit the transfer of free NF-ⲕB to the nucleus.
Before delving into some active technologies, however, it is important to also detail sirtuins (arguably the “star” of modern anti-aging research).
Sirtuins
Sirtuins (SIRT) are a hot topic in anti-aging research. This family of proteins modifies epigenetic expression through histone deacetylation. Histones are proteins around which DNA is wrapped as a regulation mechanism, among other functions (such as post translational modification through transferring ADP ribose moieties to proteins).
SIRT expression has been associated with prolonged longevity in human cell cultures. SIRT1 activity in skin keratinocytes decreases upon UV radiation, consistent with extrinsic aging, and SIRT reduces cellular senescence and apoptosis.3 One of the ways SIRTs achieve these anti-aging feats is by inhibiting NF-ⲕB activity, which has several downstream effects detailed in Figure 3, below.3 To add to this complexity, some expression of SIRT6 inhibits NF-ⲕB; however, overexpression of SIRT6 may also increase TNFα activity, which promotes NF-ⲕB. This underscores the need for precise control of active technologies.
SIRT and Niacinamide
Niacinamide provides a great example of the complexity of these systems. In Figure 4, below, NAM represents niacinamide as an inhibitor of SIRT activity. This might seem counterintuitive because a known anti-aging active (niacinamide) is inhibiting an anti-aging pathway. However, niacinamide is also metabolized to NAD+ in active cells through a salvage process and many SIRTs rely on NAD+ for their activity.4 Therefore, niacinamide might increase SIRT activity long term despite an initial inhibitory effect.
This is a cycle. SIRTs break down NAD+ into niacinamide (negative feedback loop) but niacinamide is also recycled back into NAD+. Further research must be carried out to confirm the impact of topical niacinamide on this balance, but it is an apt demonstration of how several cellular processes intertwine to create the clinically relevant phenotypes we perceive; no single pathway “rules all.” Other common actives that evidently increase SIRT activity are resveratrol (SIRT1 inducer) and bakuchiol (retinol alternative).3, 5
Plant Stem Cell Technology
To delve now into some active technologies, consider stem cells, which are well-known for their use in regenerative medicine and ability to differentiate into many cell types. Plant stem cells coincide with today’s broader cosmetic trend for sustainable technologies that are environmentally friendly, well-accepted by consumers and clinically proven.6
Plant stem cells can be upcycled from discarded plant material, grown in bioreactors to avoid seasonal dependence (unlike traditional extracts), and stimulated to produce specific actives through a process called elicitation.6 Elicitation entails the use of a stressor on the stem cell that incentivizes it to produce more metabolites with targeted benefits.7 After elicitation, the cell is typically lysed and sold as a blend containing all the compartments of the cell (including the extra metabolites).
Circling back to NF-ⲕB, one can envision a plant stem cell solution with enhanced metabolites that inhibits NF-ⲕB activity. Bianconi, et al., for example, demonstrated exactly this using red carrot stem cells cultured in vitro that underwent elicitation and successfully inhibited NF-ⲕB.6 In these tests, the cells were maintained in Gamborg’s B5 medium with 25 g/L sucrose and 3 mg/L naphthalenacetic acid, in a pH adjusted to 5.5 and incubated at 25°C in the dark. After 12 days of fermentation, the anthocyanin content (the target metabolite) was increased by placing the cells in a higher volume (50 L) bioreactor containing Gamborg’s B5 liquid medium with a higher sucrose concentration (40 g/L). The resultant cells were passed through a filter to remove the medium, homogenized and dried to form a lysate blend.
A monocyte/macrophage cell line was used to assess the blend’s anti-inflammatory activity. These immune cells were stimulated with E. coli lipopolysaccharides to produce inflammatory markers (such as NF-ⲕB). The immune cells were then lysed and probed with antibodies for the target markers. A reconstructed human epidermis (RHE) composed of human keratinocytes on an inert polycarbon filter was also used to model the activity of the stem cell active on epidermal tissue.
The stem cell lysate had broad-base anti-inflammatory efficacy. IⲕB⍺ expression (an NF-ⲕB inhibitor) was preserved when the stimulated immune cells were exposed to the stem cell lysate, as opposed to a typical reduction in IⲕB⍺, which would free NF-ⲕB. The lysate also reduced the expression of several pro-inflammatory markers (IL-1β, TNF⍺, Cox2, etc.), which reinforces anti-inflammatory efficacy.
RHE data further supported NF-ⲕB inhibition. In this case, the lysate was dispensed in the epidermis model through two conditions: one mimicking topical administration and the other mimicking systemic administration. Topical administration significantly reduced nuclear translocation of NF-ⲕB. The lysate also showed a dose dependent increase in Vascular Endothelial Growth Factor expression, which promotes angiogenesis. This is another preferential anti-aging result, as vascular access of the epidermis declines with age due (in part) to decline of the papillary dermis (i.e., the dermal region that supplies nutrients to the epidermis). Overall, plant stem cells are a promising resource of actives with proven efficacy, provided that the manufacturing process is highly standardized and cost effective for formulation use.
Archaea
Archaea are unicellular organisms distinct from the two other domains of life: bacteria and eukaryotes (where humans are categorized). Archaea have unique characteristics and molecular processes that, like plant stem cells, have brought them into the spotlight for cosmetic use. One of these unique characteristics is their ability to survive in extreme environmental conditions (such as high heat, high salinity or low pH).
Much of this can be attributed to their membrane composition. Whereas eukaryotic membranes contain high ester content due to the traditional fatty acid structure (glycerol backbone + fatty acid tails), archaeal membranes contain ether bonds that are more resilient.8, 9 Carboxylic esters in liposomes contain a partial positive carbon atom that is sensitive to nucleophilic attack, unlike ethers. This is the premise behind archaeosomes: active delivery vehicles leveraging archaea membrane components as liposome alternatives.
Figure 5, below, demonstrates key differences between archaeosomal and liposomal properties. For one, most archaea contain dialkyl glycerol ethers (DGD) instead of carboxylic esters (as seen in the ethanolamine-containing liposome). This helps to avoid oxidative degradation of archaeosomes, thus enhancing stability and allowing for storage with oxygen.10
Archaeosomes also exhibit less fluctuation in volume or compressibility with changes in temperature, making them more temperature resilient. One way that thermophilic archaea achieve this is by dynamically increasing the cyclopentane content of their membranes to adapt to increasing temperatures.8
Archaeosomes are also remarkably stable against salt and other ionic species. In molecular dynamic simulation models, no significant change was found in archaeal lipid structure across multiple measures (membrane thickness, area, order parameters, orientation, etc.) when NaCl exposure was varied from 0.1 M to 1 M.9 Only a slight reduction in membrane area was observed with 4 M NaCl concentration due to the formation of salt bridges.
This trend holds true for pH as well. Archaea that thrive in low pH environments (such as genus Sulfolobus) can maintain a cytoplasmic pH of 6.5 despite an external pH of 2-4.8 From a formulation standpoint, these properties can be utilized to greatly enhance active stability against pH, heat, oxygen or salt.
Archaeosomes have thus far mainly been used to protect oral drugs against degradation by the digestive system (such as by stomach acids). However, preliminary data on the topical use of archaeosomes is promising. Archaeosomes have been found capable of delivering methylene blue to hydrated rat skin in vitro and slowing the release of a drug for cancer treatment.8, 11 In multiple studies, a mix of conventional liposomal components (cholesterol) with archaea components (tetraether lipids) seemed to produce the best results.8, 11
Like plant stem cells, other products of archaea metabolic activity also have utility in personal care. Squillaci, et al., were able to extract carotenoids (tetraterpene pigments) from the halophilic (or salt loving) archaea Haloterrigana turkmenica.10, 12 The antioxidant capabilities of the carotenoids exceeded common antioxidants (BHT, ascorbic acid and alpha-tocopherol) in a DPPH analysis when used at a concentration of 7 µg/mL. Another group of researchers similarly tested the properties of exopolysaccharides (high molecular weight polymers) secreted from Haloterrigana turkmenica.10, 13 In this case, the exopolysaccharides were exceptional at emulsifying sunflower oil and olive oil in water, but not aromatic hydrocarbons or mineral oil. Additionally, the exopolysaccharides had superior moisture retention over hyaluronic acid in a 72-hour desiccator test, although they had inferior moisture absorption at 81% relative humidity.
Overall, preliminary information on the use of archaea in personal care is encouraging. Potential avenues of future research include further exploring topical administration and immunological interactions of archaea components in humans.
Bacteriophages
Bacteriophages are another emerging product category in cosmetics. These viruses are completely safe to humans but destroy targeted bacteria. Bacteriophages are effective microbiome-rebalancing solutions due to their high specificity, ability to circumvent antibiotic resistance, clean manufacturing methods and safety profile.14, 15
Bacteriophages are approved by the U.S. Food and Drug Administration as food additives against pathogens. They also already reside on human skin and are essential for optimal skin health;14, 15 for example, differences in phage composition between individuals have been linked to psoriasis prevalence.15 Countless therapeutic applications are being researched, with many using a cocktail of bacteriophages to minimize phage resistance.
Pelyuntha, et al., formulated Staphylococcus bacteriophages in a skin care serum.14 The phages were all naturally derived from wastewater or soil and then exposed to several Staphylococcus strains. The most effective phage was able to reduce S. aureus count by 2 log units (100×) and S. capitis by 4.1 log units (~10,000×) after six hours of treatment. After 24 hours, the lytic activity surged to more than 8 log units in both cases (99.999999% eradication of bacteria).
To assess stability, the researchers formulated the phages into a serum additionally containing deionized water (93.675%), hydroxyethyl cellulose (1.2%), glycerin (3%) and salidant DMH (0.125%). The data results are visualized in Figure 6, below. Phage viability only slightly decreased after 90 days of storage at 4°C and 25°C. Stability faltered more substantially after 60 days of storage at 37°C. The choice of preservative also influences phage viability; in the same study concentrations of 0.25%+ v/v salidant DMH nullified phage activity.
Pseudomonas acnes, the bacteria implicated in acne, is one target of recent developments in bacteriophage technology. In the pilosebaceous units of healthy skin samples, a 1:120 ratio of bacteriophage:P. acnes has been reported.16 A cocktail approach for anti-acne bacteriophage technology is challenging, however, due to the low diversity of P. acnes phages in humans.16
To isolate and characterize phages that could lyse P. acnes, Brown, et al., formulated P. acnes bacteriophages into cetomacrogol creams and tested their antimicrobial efficacy.17 The creams did not lyse other bacteria of genus Pseudomonas and halted P. acnes growth. Lytic activity remained beyond 90 days when stored away from light and at 4°C. Storage at 45°C and 25°C with light exposure reduced lytic activity span to 14 days and 21 days, respectively.
More advanced formulation or storage approaches may be necessary to enhance the stability of phages in anti-acne applications. Still, while not without challenges, the diverse utility, potent efficacy and attractive sourcing conditions of bacteriophages make them a promising prospect for product development.
Conclusion
Innovation is the engine that propels humanity forward. With each passing generation, technological innovation grows exponentially. Plant stem cells, archaea and bacteriophages are only some of the most promising developments to democratize beauty using biotechnology.
Science has only scratched the surface to uncover the inner machinations of skin physiology with the current knowledge of inflammaging and cellular signaling. As we stand upon the shoulders of giants and inherit the ingenuities of generations past, it is now our responsibility to bear Prometheus’ flame. What futures will we illuminate?
References
1. Pilkington, S.M., Bulfone-Paus, S., Griffiths, C.E.M and Watson, R.E.B. (2021). Inflammaging and the skin. J Invest Dermatol. Available at https://pubmed.ncbi.nlm.nih.gov/33358020/
2. García-García, V.A., Alameda, J.P., Page, A. and Casanova, M.L. (2021). Role of NF-κB in aging and age-related diseases: Lessons from genetically modified mouse models. MDPI. Available at https://www.mdpi.com/2073-4409/10/8/1906
3. Serravallo, M., Jagdeo, J., Glick, S.A., Siegel, D.M. and Brody, N.I. (2013). Sirtuins in dermatology: Applications for future research and therapeutics. Arch Dermatol Res. Available at https://pubmed.ncbi.nlm.nih.gov/23377138/
4. Hwang, E.S. and Song, S.B. (2017). Nicotinamide is an inhibitor of SIRT1 in vitro, but can be a stimulator in cells. Cellular and Molecular Life Sciences. Available at https://link.springer.com/article/10.1007/s00018-017-2527-8
5. Sousa, C. and Mendes, A.F. (2022). Monoterpenes as sirtuin-1 activators: Therapeutic potential in aging and related diseases. Biomolecules. Available at https://www.mdpi.com/2218-273X/12/7/921
6. Bianconi, M., Ceriotti, L., … Guzzo, F., et al. (2020). Red carrot cells cultured in vitro are effective, stable and safe ingredients for skin care, nutraceutical and food applications. Front Bioeng Biotechnol. Available at https://pubmed.ncbi.nlm.nih.gov/33195137/
7. Narayani, M.S. and Srivastava, S. (2017). Elicitation: A stimulation of stress in in vitro plant cell/tissue cultures for enhancement of secondary metabolite production. Phytochem Rev. Available at https://link.springer.com/article/10.1007/s11101-017-9534-0
8. Rastädter, K., Wurm, D.J., Spadiut, O. and Quehenberger, J. (2020). The cell membrane of Sulfolobus spp. — Homeoviscous adaption and biotechnological applications. Int J Mol Sci. Available at https://pubmed.ncbi.nlm.nih.gov/32486295/
9. Shinoda, K., Shinoda, W. and Mikami, M. (2007). Molecular dynamics simulation of an archaeal lipid bilayer with sodium chloride. Physical Chemistry Chemical Physics. Available at https://pubs.rsc.org/en/content/articlelanding/2007/cp/b611543h/unauth
10. Aparici-Carratalá, D., Esclapez, J., Bautista, V., Bonete, M. and Camacho, M. (2023). Archaea: Current and potential biotechnological applications. Res Microbiol. Available at https://pubmed.ncbi.nlm.nih.gov/37196775/
11. Moghimipour, E., Kargar, M., Ramezani, Z. and Handali, S. (2013). The potent in vitro skin permeation of archaeosome made from lipids extracted of Sulfolobus acidocaldarius. Archaea. Available at https://pubmed.ncbi.nlm.nih.gov/24453698/
12. Squillaci, G., Parrella, R., Carbone, V., Minasi, P., La Cara, F. and Morana, A. (2017). Carotenoids from the extreme halophilic archaeon Haloterrigena turkmenica: Identification and antioxidant activity. Extremophiles. Available at https://pubmed.ncbi.nlm.nih.gov/28803263/
13. Squillaci, G., Finamore, R., … Morana, A. (2015). Production and properties of an exopolysaccharide synthesized by the extreme halophilic archaeon Haloterrigena turkmenica. Appl Microbiol Biotech. Available at https://pubmed.ncbi.nlm.nih.gov/26403921/
14. Pelyuntha, W., Yingkajorn, M., Narkpao, T., Saeaui, S., Promkuljan, K. and Vongkamjan, K. (2023). Evaluation of the effectiveness of Staphylococcus phages in a skin care serum against Staphylococcus spp. Cosmetics. Available at https://www.mdpi.com/2079-9284/10/6/156/review_report
15. Wang, H., Chan, H.H., Ni, M.Y., Lam, W.W., Chan, W.M.M. and Pang, H. (2019). Bacteriophage of the skin microbiome in patients with psoriasis and healthy family controls. J Invest Dermatol. Available at https://pubmed.ncbi.nlm.nih.gov/31247199/
16. Castillo, D.E., Nanda, S. and Keri, J.E. (2018). Propionibacterium (cutibacterium) acnes bacteriophage therapy in acne: Current evidence and future perspectives. Dermatol Ther (Heidelb). Available at https://pubmed.ncbi.nlm.nih.gov/30539425/
17. Brown, T.L., Petrovski, S., Dyson, Z.A., Seviour, R. and Tucci, J. (2016). The formulation of bacteriophage in a semi solid preparation for control of Propionibacterium acnes growth. Public Library of Science (PLoS). Available at https://pubmed.ncbi.nlm.nih.gov/26964063/